COUNTERION TRIPLE LAYER FOR
EXPLAINING STIRRING AND
TEMPERATURE EFFECTS ON pH
MEASUREMENTS
K. L. CHENG
Department of Chemistry,
University of Missouri-Kansas
City, Kansas City, Missouri
64110
Abstract
This paper reports the pH glass
electrode potential based on the
double capacitor theory and a
new, novel concept of mobile
countertion triple layer next to
the double layer to explain the
electrode potential changes
caused by stirring and
temperature.
This mobile triple layer
behaving like a rubber band may
be removed by stirring or
agitation and temperature and
returned after stopping stirring
or lowering temperature.
Stirring and temperature affect
differently the potential of the
pH and SCE electrodes for their
different mechanisms. Contrary
to the past, results reported
here were obtained from the
experiments of glass electrode
and SCE in two separate beakers
connected with a conducting wire
so that stirring one electrode
while keeping the other
unstirred. There is a weak
charge attraction between the
double and triple layers, not a
strong covalent bonding for
complexation so that the latter
can be removed by stirring or
temperature. In considering the
present results and the new
concept, the Boltzmann equation
has been modified to include the
net charge density and the
triple layer potential. The
counterion triple layer concept
will have a tremendous impact on
understanding the interface
structure and properties and
stimulate further studies and
applications for ISE.
Introduction
Though the effects of stirring
and temperature on the pH glass
electrode potential have been
known for a long time and
discussed in literature (1), we
have found conflicting
recommendations on stirring in
the pH measurements (4,5).
Ineffective efforts for
compensating the stirring and
temperature effects have also
been suggested because the past
researchers did not understand
their fundamental mechanisms.
Although the double capacitor
theory for the pH glass
electrode potential has been
proposed more than a decade ago,
and ignored by most quantitative
textbooks which still describe
the redox and Nernst equation
for the ion selective electrodes
(ISE) (6,7) instead of the
capacitor and the Boltzmann
equation (6). Many
electroanalytical chemists still
believe the speculative redox
reactions and mechanisms for the
common reference electrodes, as
a result, the stirring and
temperature effects cannot be
properly explained by the Nernst
equation (7,8). This paper will
demonstrate the important role
of correct conception and
mechanism for explanation of
electrode phenomena. Hope this
paper will stimulate
electroanalytical chemists to be
open-minded and encourage new
challenges.
Experimental
A
Corning pH/ion meter 150 was
used for pH and potential
measurements. A magnetic stirrer
and Branson ultrasonic cleaner
B-12, 50/60 Hz were used for
agitation. In the study of
stirring effect on pH glass
electrode and SCE reference
electrode, putting both
electrodes in two separate
beakers containing the same 50
mL solution. The two solutions
were connected with an insulated
conducting wire. For studying
the stirring effect, one beaker
was stirred while the other
unstirred.
Results and
discussion
Application of a pressure in an
electrolyte solution gives rise
to a potential difference and
corresponding electric field.
This is the phenomenon commonly
called streaming potential (2,3)
which has been applied to the
stirring effect (2,3).
F = P (
z
/ k(10
-6 ) ) (1)
where P is the pressure in mm Hg
and (F is the zeta potential in
mV. The streaming potential has
been applied in the literature
with a capillary mode and may
not fit into the current
non-capillary mode. It seems
that the streaming potential
equation cannot be applied to
the commonly stirring effect on
glass and SCE electrodes.
The
double layer concept has been
widely discussed, a new
couonterion triple layer concept
is suggested here as shown in
Fig. 1. Except the adsorbed ions
in the inner layer are not in
the hydrated form, the ions in
the double and triple layers are
in the hydrated form (not shown
in Fig. 1). This triple layer
containing counterions is
attracted to the double layer by
the charge attraction force, not
the covalent bonding
complexation force. This is
evidenced by its mobile
characteristics. Its thickness
is controlled by its counterion
concentration, temperature,
solvent, and other factors.
Because of its characteristics,
the present results suggest the
presence of the counterion
triple layer concept. It can
explain the stirring and
temperature effects on ISE and
may be applied to other cases.
The stirring effect results are
shown in Figs. 2-6. As reported
previously (6,7), as a
capacitor, in an acidic solution
the pH glass electrode is
adsorbed with H+ and
in a basic solution it is
adsorbed with OH-
showing a positive or negative
potential, respectively (9). Its
potential may be derived by the
modified Boltzmann equation
(10). In an unstirred solution,
the electrode potential is
E
unstirred = E i
+ E d - E cit
(2)
where E i , E d
and E cit are
inner layer potential, double
layer potential, and counterion
triple layer potential,
respectively. In a stirred
solution, the electrode
potential will be
E
stirred = E i +
E d (3)
Then
Ecit = E stirred
- E unstirred
(4)
Stirring actually enhances the
electrode potential by removing
the counterion triple layer
effect. The difference between
the stirred potential and the
unstirred potential is the
counterion triple layer
potential which is illustrated
in Figs. 2-5. In an acidic
solution, the electrode is
positively charged, at the
interface, the counterion anions
will compensate the electrode
potential (or charge density)
resulting in a less positive
potential. In a basic solution,
the electrode is negatively
charged, at the interface, the
counterion cations will
compensate the electrode
potential resulkting in a less
negative potential, The
stirrignand temperature effects
as a function of pH are in a
linear relationship as shown in
Figs. 3- 4. When the solution pH
went to more acid the stirred
potential increased to more
positive and pH went to more
basic, the stirring potential
moved to more negative. As shown
in Fig. 5, the stirring effect
is reasonably repeatable. At pH
10, the stirring effect showed
somewhat unevenly. This might be
due to somewhat different
adsorption forms for H+
and OH- ions.
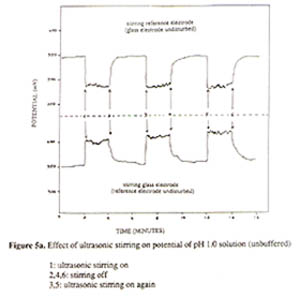
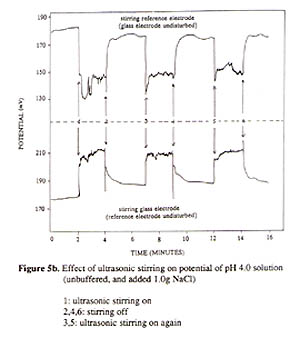

A
special attention should be made
that in comparing the stirring
effects of glass and SCE
electrodes, they had an opposite
effect (Fig. 4). The intersects
for the two curves in Figs. 2
and 4 is not at pH 7, instead,
at approximately pH 6.5. This is
in agreement with the
isoelectric point of glass at
around 6.5 ( 7 ). In the
literature it has been
mistakenly assumed that the
isoelectric point of pH glass is
7 ( 4, 12, 13 ). One must
realize that the stirring effect
on the two electrodes is not the
same, having different
directions. So it is difficulty
to study and compensate the
stirring effect for various pH
values while the two electrodes
are in the same solution or
using the combination electrode.
It was possible for us to study
the stirring and temperature
effects by putting the two
electrodes in two separate
beakers.
Results of the stirring and
temperature effects on the glass
electrode and SCE are shown in
Fig. 3 indicating that stirring
and temperature could remove the
effect against the electrode
potential providing that the
reference electrode potential is
kept constant.
The
stirring effect on the SCE and
glass electrode at different
temperatures are quite different
(Figs. 6a and 6b). The
ultrasonic stirring of the SCE
at pH 10 gave very unstable
potentials (6b) because the Hg
2Cl2 is
relatively separated far away
from the sample solution. The
SCE is negatively charged due to
adsorption of Cl-. The stirring
would remove the counterion
triple layer of cations, but
also the part of KCl so that the
SCE went to more positive. The
junction potential at the tiny
holes of the SCE might have had
discontinuous changes of KCl
concentration, non-equilibrium
situation. The potential changed
to more positive indicates the
removal of chloride anions from
the KCl solution at the
junction. At higher temperatures
the junction holes become larger
making stirring easier to move
away more chloride anions
diluting the KCl concentration.
So the resulting SCE potential
became more positive and
relatively stable. This also
confirms that SCE behaves as a
capacitor of Cl- ISE,
not a redox electrode. It is
desirable to develop a stable
reference electrode resisting
environmental changes.

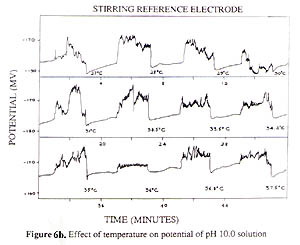
Both Nernst and Boltzmann
equations do not explain the
stirring and temperature effect
mechanisms, they only show the
temperature slope. The modified
Boltzmann equation includes the
net charge density (10). Now, in
consideration of the presence of
counterion triple layer, it may
be further modified,
E
unstirred = 2.3 kT/ (Z ?e)
log (Ni/No)
- E cit (5)
where Z ?is the net charge
density for the zwitterionic
electrode surface and E cit
is the counterion triple layer
potential. One should remember
that the Boltzmann equation only
provides the electrode potential
without considering the
counterion triple layer effect
which always exists in a
practical, unstirred
electrolytic solution. In other
words, the potential calculated
from the Boltzmann equation
based on the electrode surface
charge density is actually the
potential of stirred solution
without the counterion triple
layer effect.
The
counterion triple effect differs
from other triple layer models.
For instance, Hayes (11)
described his model as surface
complexation model (SCMS) that
are used to describe the
partition of inorganic metal
ions between mineral and aqueous
phases. These models are based
on the premise that minerals
have surface functional groups
capable of forming complexes
with ions in the interface
region. Our triple layer model
is mobile counterion phase with
an opposite charges to the
double layer, not related to
metal ions for stable mineral
complex formation.
Conclusion
A
counterion triple layer concept
has been proposed for explaining
the stirring and temperature
effects on pH glass electrode
potential measurements which
have not been adequately
explained. It may also be
applied to other cases. The pH
glass and the SCE reference
electrodes are considered as
capacitors, their potentials are
related to the capacitance
potentials, E = q/C. This
counterion triple layer is the
one containing counterions next
to the double layer and may be
removed by stirring and
temperature. It is mobile like a
rubber band, returning to the
original potential after
stopping stirring or lowering
the temperature. The Boltzmann
equation has been modified to
include the net charge and
counterion triple layer effects.
Removal of the triple layer
enhances the electrode
potential. Experiments using
both glass electrode and SCE in
two separate beakers made it
possible to obtain the results
reported here, because the two
electrodes have different
effects on potentials at
different pH values The new
concept seems to explain well
the stirring and temperature
effects on the pH measurements.
Its applications to other cases
are anticipated. Any concept,
theory, or mechanism must be
able to explain phenomena
correctly, otherwise, they
should be modified or discarded.
References
[1]
Ching-I Huang, Hsuan Jung Huang,
K. L. Cheng, Chapter in T. F.
Yen, et al (editors), Advances
in the Applications of
Membrane-Mimetic Chemistry.
Plenum Press, New York, (1994),
pp 227-240.
[2]. J. O’M Bockris and A. K. N.
Reddy, Modern Electrochemistry.
Vol. 2, Plenum Press, New
York,(1977).
[3]
D. R. Crow, Principles and
Applications of
Electrochemistry. Chapman and
Hall, New York, (1988), 3rd
edition.
[4]
"Orion Research Analytical
Analysis Guide" , 4th ed.
Cambridge, Ma. October (1972).
[5]
R. A. Durst, ed. Ion Selective
Electrodes. National Bureau of
Standards, Special Publ., 314,
Washington, D. C. (1969).
[6]
K. L. Cheng, Microchem. J., 42,
(1990) 5.
[7]
K. L. Cheng, pH glass electrode
and its mechanism in
Electrochemistry, Past and
Present. J. T. Stock and M. V.
Orna, (Eds.). ACS Symposium
Series 390, pp. 286-(1989). The
Second Nernst Hiatus, paper
presented at the Pittsburgh
Conference, 1992.
[8]
Khalid R. Temsamani, K. L.
Cheng, Challenges to
conventional redox mechanisms of
calomel and Ag/AgCl reference
electrodes. Paper presented at
the Colloid and Surface Chem.
Div. , 215th ACS national
Meeting (1998). Dallas, TX.
[9]
K. L. Cheng, H. Z. Song, S. X. R
Yang, J. Chem. Soc. Commun.,
(1988) 1333.
[10] K. L. Cheng, Microchem. J.,
59 (1998) 457.
[11] K. F. Hayes, Equilibrium,
spectroscopic, and kinetic
studies of ion adsorption at the
oxide/aqueous interface.
Dissertation, Stanford
University, Stanford, Ca. (
1987).
[12] "Practice and Theory of pH
Measurement", Ingold, Ordorf,
Switzerland (1989).
[13] "Handbook of Electrode
Technology", Orion Research,
Cambridge, Ma. (1982). |